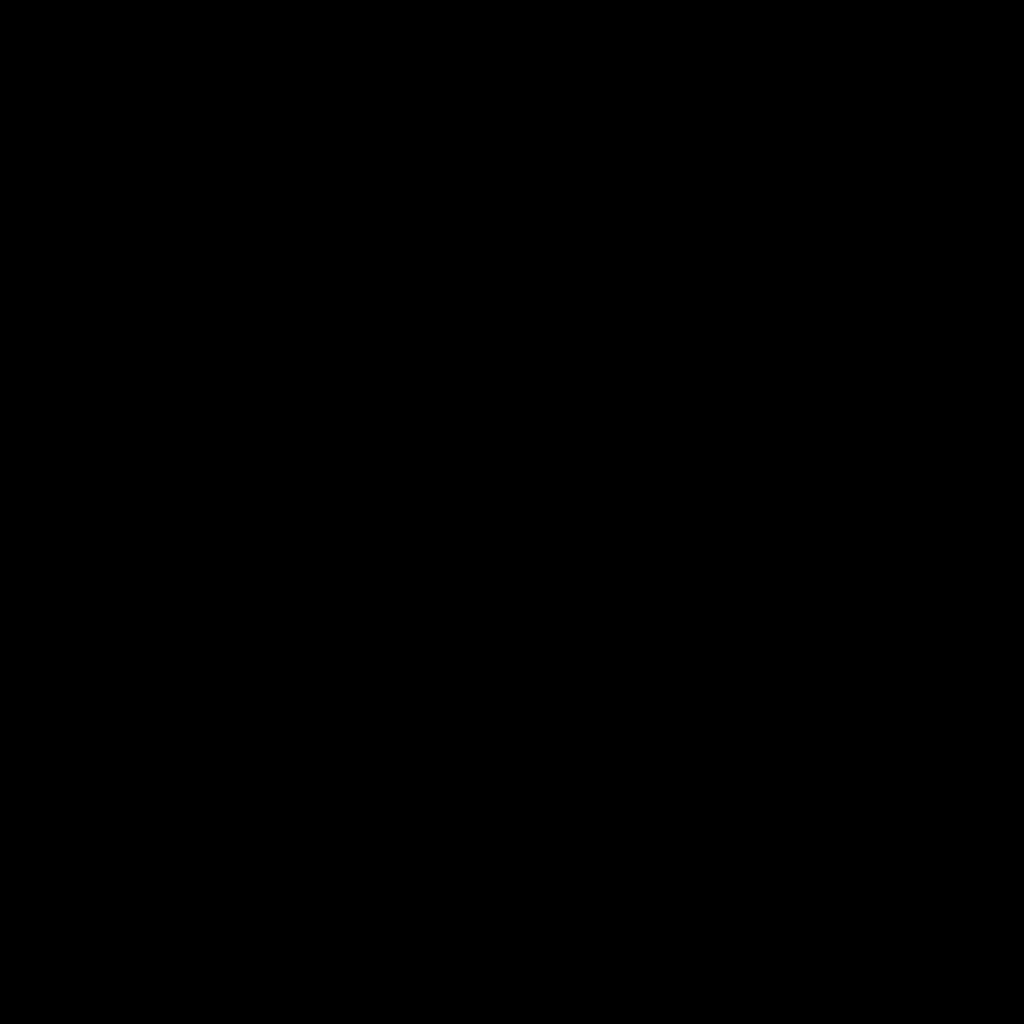
Unraveling Protein Structure and Dynamics: A Deep Dive into Hydrogen Deuterium Exchange Mass Spectrometry (HDX-MS)
Hydrogen Deuterium Exchange Mass Spectrometry (HDX-MS) has emerged as a powerful biophysical technique for characterizing protein structure, dynamics, and interactions. This method leverages the inherent isotopic exchange of labile hydrogen atoms in proteins with deuterium, providing insights into solvent accessibility and conformational changes at various levels of structural organization. This comprehensive exploration will delve into the principles, methodologies, data analysis, and diverse applications of HDX-MS in modern biological research.
Principles of Hydrogen Deuterium Exchange
The foundation of HDX-MS rests on the principle of isotopic exchange. Labile hydrogen atoms in proteins, primarily those attached to amide nitrogens in the peptide backbone and side chains of certain amino acids (e.g., Ser, Thr, Tyr), readily exchange with deuterium atoms present in deuterated water (D2O). The rate of this exchange is highly sensitive to the protein’s environment. Hydrogen atoms in regions with high solvent accessibility exchange rapidly, while those buried within the protein core or involved in strong hydrogen bonds exchange much more slowly. This differential exchange rate provides a valuable window into the protein’s structural organization and dynamics.
- Solvent Accessibility: Exposed residues readily exchange deuterium, while buried residues exchange slowly.
- Hydrogen Bonding: Strong hydrogen bonds hinder deuterium exchange, resulting in slower exchange rates.
- Conformational Dynamics: Regions undergoing conformational changes often exhibit altered exchange rates, revealing dynamic processes.
- Protein Interactions: Upon binding to a ligand or another protein, the exchange rates of specific regions can change, reflecting the interaction interface.
Methodology of HDX-MS
The HDX-MS workflow typically consists of several key steps:
- Protein Preparation: Purified protein is prepared in appropriate buffer conditions.
- Deuterium Exchange: The protein is transferred to D2O, initiating the exchange process. The exchange is typically quenched after a specific time interval to control the extent of deuterium incorporation.
- Proteolytic Digestion: To reduce the complexity and improve mass spectrometric analysis, the protein is digested using an enzyme such as pepsin, which efficiently cleaves proteins under low pH and temperature conditions. Rapid digestion minimizes back-exchange (the reversion of deuterium to hydrogen) during the process.
- Chromatographic Separation: The resulting peptide fragments are separated by liquid chromatography (LC), typically using a reversed-phase HPLC system.
- Mass Spectrometry Analysis: The separated peptides are analyzed by mass spectrometry (MS), enabling the determination of their mass-to-charge ratios (m/z). The difference in mass between the deuterated and undeuterated peptides provides a measure of the extent of deuterium incorporation.
- Data Analysis: Sophisticated software packages are used to analyze the mass spectrometry data. The deuterium uptake is determined for each peptide, providing information about the solvent accessibility and dynamics of the corresponding protein region.
Data Analysis and Interpretation
HDX-MS data analysis requires specialized software and expertise. The core aim is to determine the deuterium uptake for each peptide and interpret this in the context of the protein’s structure and function. Several key aspects of data analysis are:
- Deuterium Incorporation Calculation: Software calculates the average number of deuterium atoms incorporated into each peptide.
- Data Normalization and Correction: Corrections are often applied to account for back-exchange and other experimental variations.
- Mapping to Protein Structure: Deuterium incorporation data is mapped onto the protein’s three-dimensional structure (if available) to visualize regions with different exchange rates.
- Statistical Analysis: Statistical methods are employed to identify significant differences in deuterium uptake between different conditions (e.g., comparing apo and holo protein states).
- Data Visualization: The results are often presented visually using heatmaps, graphs, and other graphical representations to facilitate interpretation.
Applications of HDX-MS
HDX-MS has broad applicability in various fields of biological research:
- Protein Structure Determination: HDX-MS can complement other structural techniques such as X-ray crystallography and NMR spectroscopy, providing information about dynamic regions and conformational changes that are often difficult to capture using traditional methods.
- Protein Folding and Unfolding Studies: HDX-MS can monitor the unfolding of proteins under various stress conditions (e.g., temperature, pH, denaturants), providing insights into protein stability and folding pathways.
- Protein-Ligand Interactions: HDX-MS is used to study the interactions between proteins and small molecules, such as drugs or substrates. Changes in deuterium uptake upon ligand binding can reveal the binding site and the conformational changes induced by binding.
- Protein-Protein Interactions: HDX-MS can identify the binding interfaces in protein-protein complexes, shedding light on the mechanisms of protein-protein interactions.
- Enzyme Mechanisms: HDX-MS can investigate the conformational changes that occur during enzyme catalysis, helping elucidate enzyme mechanisms.
- Post-Translational Modifications: HDX-MS can provide information on how post-translational modifications affect protein structure and dynamics.
- Membrane Protein Studies: HDX-MS is being increasingly applied to study membrane proteins, a challenging area for other structural techniques. The method can provide insights into the membrane protein’s conformation and its interactions with lipids and other molecules.
Advantages and Limitations of HDX-MS
HDX-MS offers several advantages over other biophysical techniques:
- Sensitivity to subtle conformational changes: HDX-MS can detect even subtle changes in protein structure and dynamics.
- No need for protein crystallization: HDX-MS can be performed on proteins in solution, eliminating the need for crystallization, which can be challenging for some proteins.
- Information on protein dynamics: HDX-MS provides insights into the dynamics of proteins, which are often difficult to obtain using other methods.
- Ability to study large protein complexes: HDX-MS can be used to study large protein complexes.
However, HDX-MS also has some limitations:
- Back-exchange: Back-exchange can complicate the interpretation of data, requiring careful experimental design and data correction.
- Peptide coverage: Not all peptides can be observed in HDX-MS experiments, potentially leading to incomplete coverage of the protein.
- Complexity of data analysis: HDX-MS data analysis can be complex, requiring specialized software and expertise.
- Cost and instrumentation: HDX-MS requires specialized equipment and expertise, which can be expensive.
Future Directions of HDX-MS
HDX-MS is a rapidly evolving field, with ongoing developments in instrumentation, methodology, and data analysis. Future directions include:
- Improved instrumentation: Advancements in mass spectrometry technology will continue to improve the sensitivity and speed of HDX-MS experiments.
- Development of new data analysis methods: More sophisticated data analysis methods are needed to fully exploit the large datasets generated by HDX-MS.
- Expansion of applications: HDX-MS will continue to find new applications in diverse areas of biological research.
- Integration with other techniques: The integration of HDX-MS with other techniques, such as NMR and cryo-EM, will provide a more comprehensive understanding of protein structure and dynamics.
- High-throughput HDX-MS: Development of higher throughput methods will accelerate the application of HDX-MS in drug discovery and other high-throughput settings.
In conclusion, HDX-MS has emerged as a powerful tool for studying protein structure, dynamics, and interactions. Its ability to provide detailed insights into solvent accessibility, conformational changes, and binding interfaces makes it a valuable complement to other biophysical techniques. As the technology continues to advance and its applications expand, HDX-MS is poised to play an increasingly important role in advancing our understanding of biological processes at the molecular level.